A Dual Band Channel Sounder Module for FR1 & FR3 Band Modelling (6.75 GHz & 16.95 GHz)
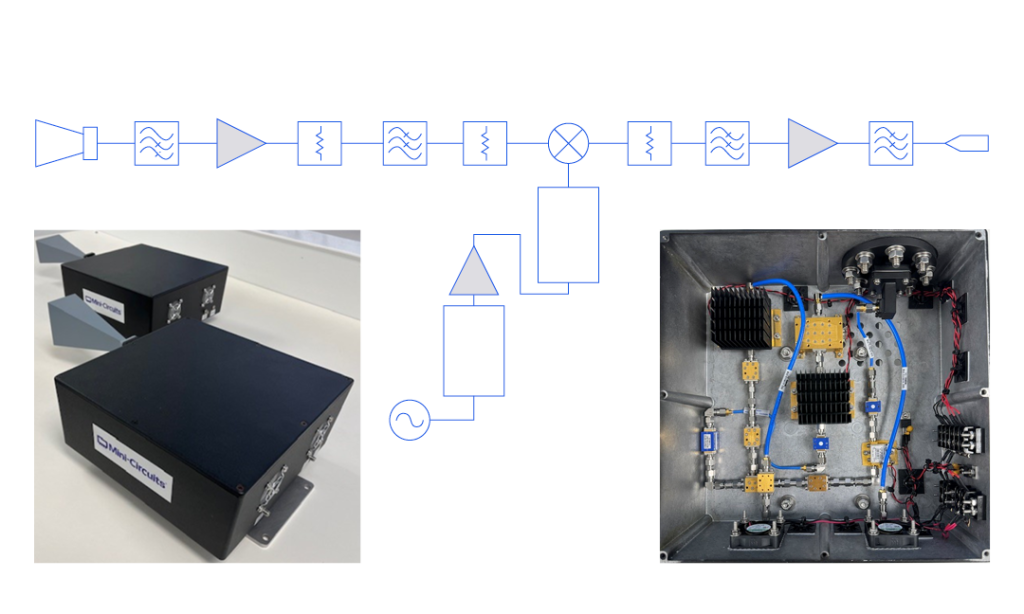
While much research has been devoted to exploring millimeter-wave bandwidths for high-data-rate wireless communications, much of the deployment of 5G to date has relied on frequencies in the sub-6 GHz (FR1) region of the spectrum. The channel capacity of the FR2 bands has been used in urban environments with high subscriber demand where infrastructure can be installed with sufficient density to compensate for the short range and poor penetration of high-frequency signals. Meanwhile, network operators still rely on lower-frequency signals for more ubiquitous coverage.
Similar desire for the data capacity and speed of millimeter-wave and sub-THz transmissions with broad network coverage and low power requirements of lower frequencies has spurred strong interest in the FR3 bands (7 to 24 GHz) as a possible “Goldilocks zone” for the next phases of 5G and 6G development.
Professor Ted Rappaport and his graduate research fellows of NYU WIRELESS in Brooklyn, New York are among the leading researchers exploring the propagation characteristics of 5G and 6G frequency bands under consideration for commercial use by the ITU and telecom industry. In 2022 Rappaport and his team visited Mini-Circuits’ facilities in Brooklyn, and Deer Park on Long Island as a test bed for their work to develop the first spatial statistical model for ultra-wideband signals above 100 GHz in a real-world factory environment.
Group Delay in RF Filters
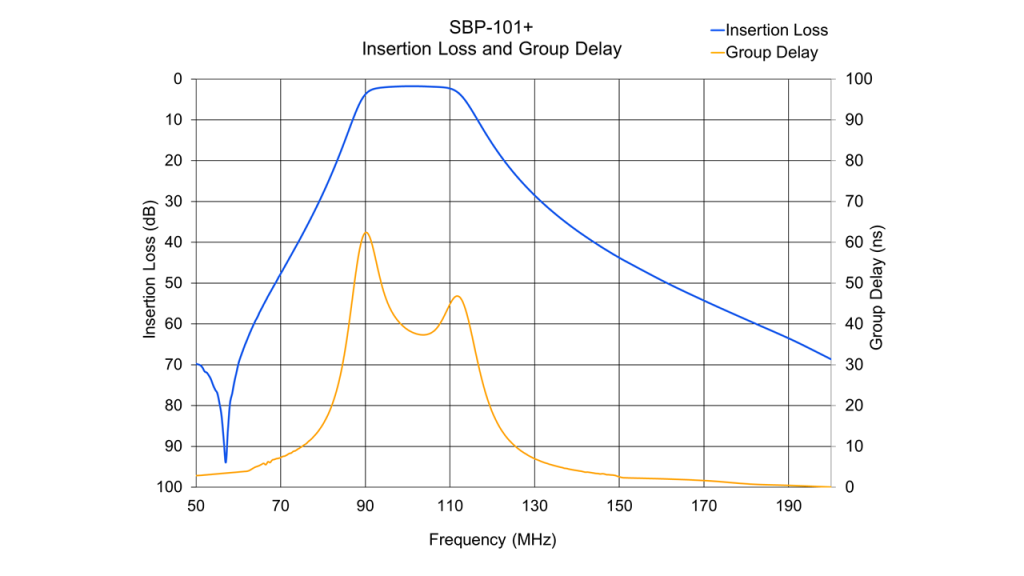
The concept of group delay as it pertains to RLC networks was first described by Harry Nyquist in 1928.1 The contributions Nyquist made to the field of Communications Theory are well known,2 and still applicable to modern day communications systems. On our way toward understanding group delay in this brief application note, we’ll start by examining phase delay. After mathematically defining phase delay, we will then continue by defining group delay. Illustrations of amplitude response, and phase and group delay response for several ideal filters of various filter topologies are shown. This app note concludes with a display of magnitude and group delay frequency response curves for two real filters designed and manufactured by Mini-Circuits, both of which have the same filter topology.
Frequency Modulation Fundamentals
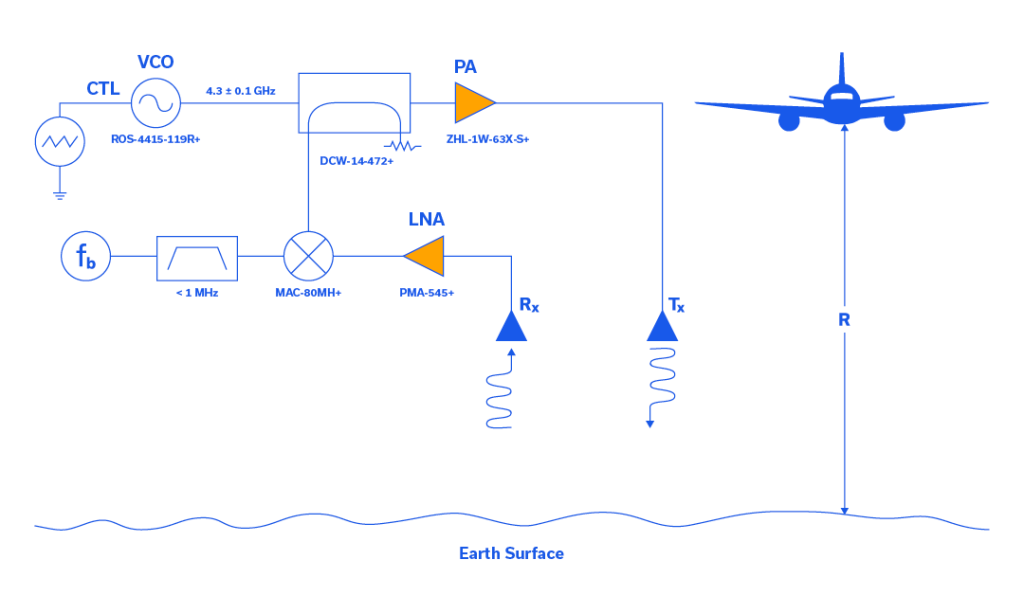
In the 1920s, many brilliant scientists applied themselves to the study of frequency modulation (FM). One of these scientists was a communications systems theorist who worked for AT&T named John Renshaw Carson. Carson performed a comprehensive analysis of FM in his 1922 paper which yielded the Carson bandwidth rule.1 Carson was so convinced that FM was not a suitable solution to the static found in AM transmission systems that he once remarked, “Static, like the poor, will always be with us.”2
Beginning in 1923, in Columbia University’s Marcellus Hartley Research Laboratory, in the basement of Philosophy Hall, a driven genius in electronic circuitry named Edwin Howard Armstrong set out to reduce static through the use of FM. After approximately 8 years of toil, Armstrong had a brainstorm and decided to challenge the assumption that the FM transmission bandwidth had to be narrow to keep noise low. After painstakingly designing this new FM system, with as many as 100 tubes spread over several tables in the laboratory, “[Armstrong] was able to prove that wideband FM made possible a drastic reduction of noise and static.”3 Armstrong was issued patent number US1941069A, which specifically addresses noise suppression in wideband FM, on December 26, 1933, along with three additional patents for FM that same day.
The Basics of Orthogonal Frequency-Division Multiplexing (OFDM)
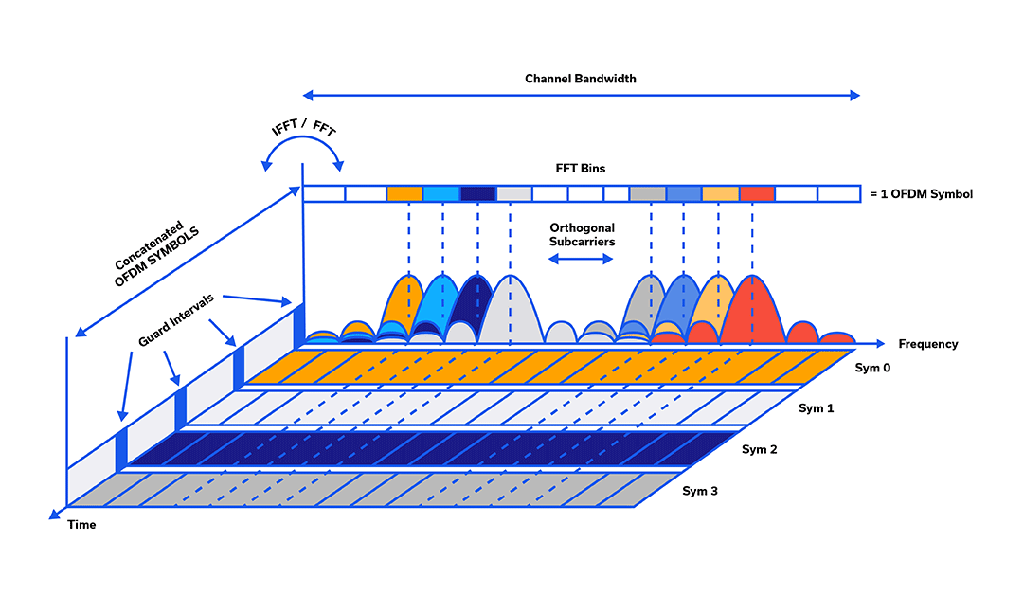
While traditional Frequency Division Multiplexing has been around for over 100 years, Orthogonal Frequency Division Multiplexing (OFDM) was first introduced by Robert W. Chang of Bell Laboratories in 1966.1,2,3,4 In OFDM, the stream of information is split between many closely-spaced, narrowband subcarriers instead of being relegated to a single wideband channel frequency.5 Single-channel modulation schemes tend to be sequential whereas, in OFDM, many bits can be sent in parallel, simultaneously, in the many subcarriers.5 So many bits can be packed onto the subcarriers simultaneously that the data rate of each subcarrier’s modulation can be much lower than that of a single-carrier architecture.
LTCC High Pass Filters for mmWave
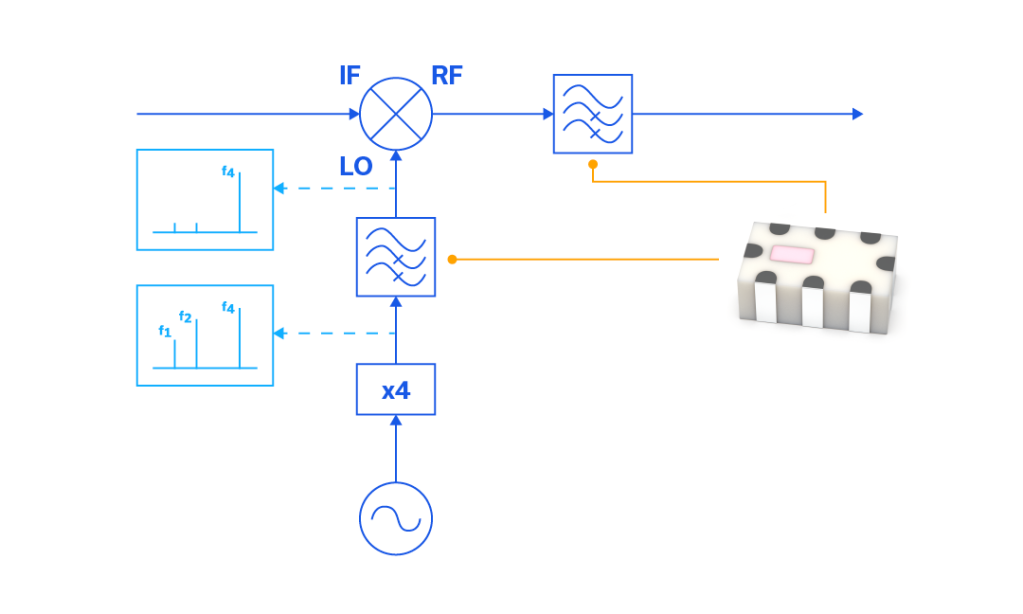
Mini-Circuits has introduced new high pass filters that achieve breakthrough performance for Q-band and lower V-band applications up to 58 GHz. The HFCQ, HFCN, and HFCV LTCC filters offer a combination of low insertion loss, high return loss, excellent rejection, small size, cost-effectiveness, repeatability, ruggedness and reliability. These filters enable designers to cover filter blocks with traditional SMT technology versus more exotic solutions. Key benefits include smaller footprint compared to stripline filters, repeatable performance suited for volume production, and robustness. The filters simplify designs for applications like 5G FR2 and satellite communications. Mini-Circuits’ latest LTCC high pass filters set a new standard for electrical performance and ease of implementation in demanding mmWave systems.
A Brief Overview of Phased Array Systems
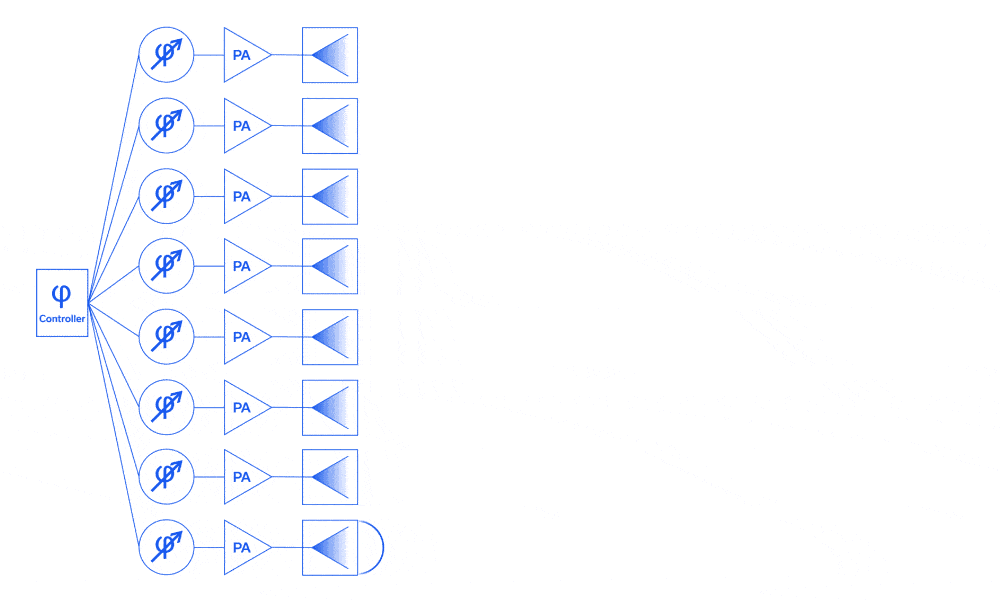
The concept of the phased array antenna system was first put into practice by German Physicist Ferdinand Braun and his assistants in the spring of 1905. In short, he and his assistants carefully controlled the excitation phase of each antenna in an array and determined that the combined effect exhibited significant directivity. In the nearly 12 decades since Braun first described the phased array antenna system, this technology has become commonplace in 4G/5G communications, electronic warfare, radar, nonlethal weaponry, and advanced imaging applications.1
This article begins with an overview of the evolution of phased array systems through history. A functional description of their basic operation in both analog and digital domains is provided, and a brief survey of common and emerging applications is given by way of example.
BOOST YOUR KNOWLEDGE: A COMPREHENSIVE GUIDE TO RF FILTERS – TYPES AND APPLICATIONS EXPLAINED
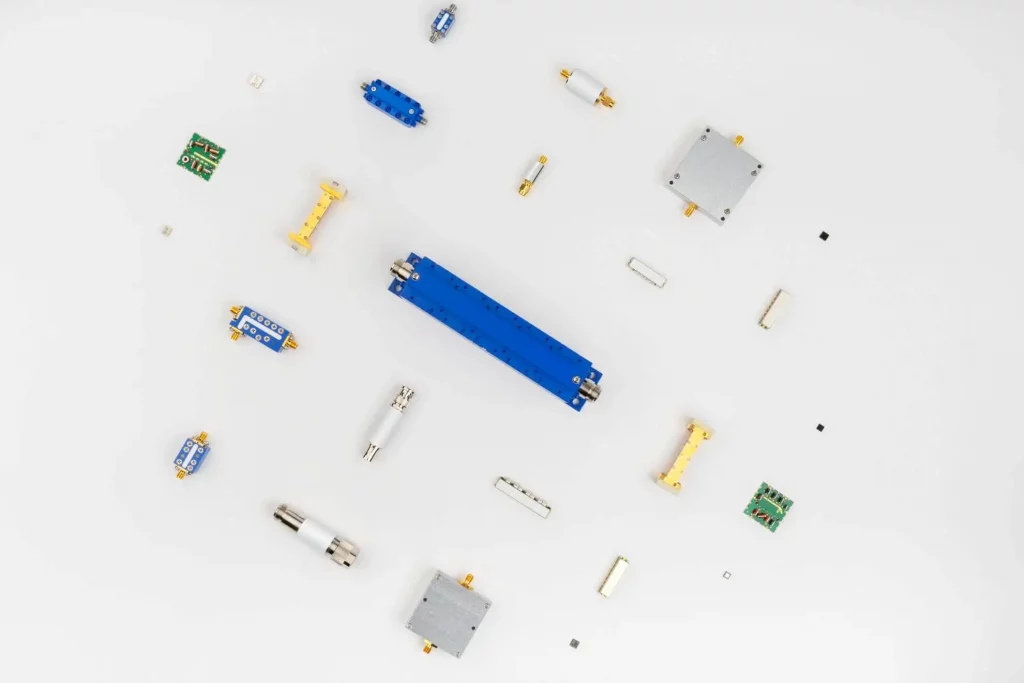
RF filters, also known as radio frequency filters, are electronic devices that are used to selectively pass or block specific frequencies of an RF signal. These devices are used in a wide range of applications, including wireless communication systems, test and measurement equipment, and RF circuit design. RF filters can also be used to remove unwanted interference or noise from a signal, or to separate different signals in a system.
There are several different types of RF filters, each with their own unique characteristics and uses. In this blog post, we will discuss the different types of RF filters and their applications.
A Primer on Quadrature Amplitude Modulation (QAM)
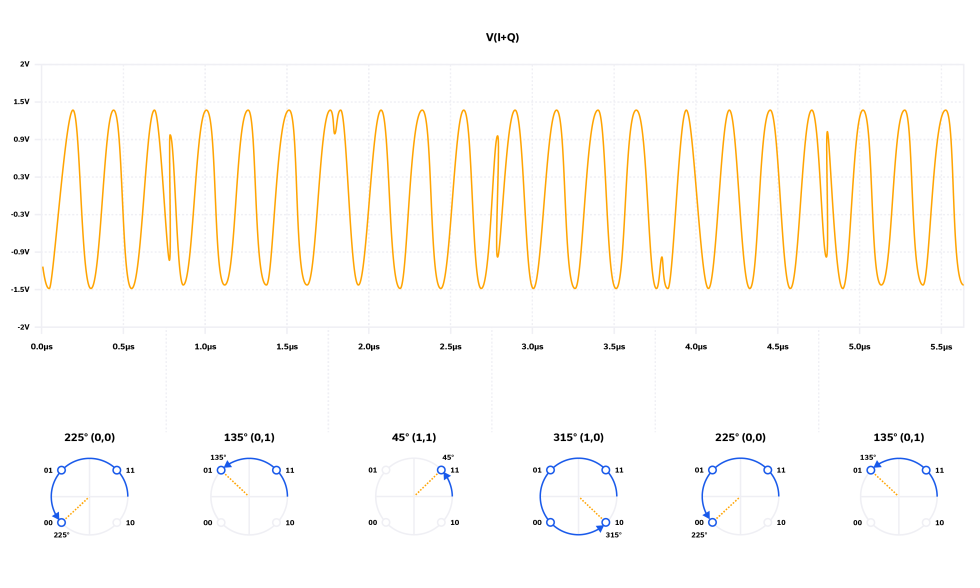
While other modulation schemes discussed in this blog series (pulse, frequency, amplitude, phase) date back to the early chapters of RF engineering history, quadrature amplitude modulation (QAM) was first described by C. R. Cahn in 19602 and evolved steadily over the next few decades. In the last 25 to 30 years, no modulation scheme has seen such widespread development and application as QAM. The technology has played a pivotal role in the industry’s ability to scale data speed and capacity with user demand by packing more data onto the carrier waveform and pushing a fixed channel bandwidth closer to Shannon’s limit. QAM modulation is used widely in cellular networks and backhaul, CATV networks and fixed wireless access points (802.11), and satellite communications to name a few. See Table 3 in Reference [3] for a more detailed list of applications.
In this article, we describe QAM using basic mathematics and illustrate how a QAM modulator operates. We introduce the concept of a constellation diagram and how it relates to the time domain plots for QAM modulation. A representative set of components is then utilized to design a functional QAM modulator by way of illustration. We conclude by describing how the QAM signal is demodulated at the receiver.
LTCC Filter Innovations Enable Next Generation Aircraft Internet Links
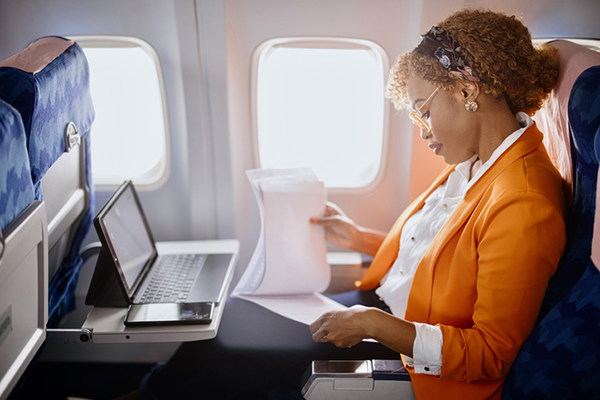
For all the headlines and personal anecdotes lamenting how commercial air travel isn’t what it used to be, there are some clear benefits enabled by recent advances in technology we might be taking for granted. One of these is in-flight internet service. Whether domestic or international, most flights now offer internet service via satellite, allowing passengers to remain connected for personal and business use throughout the majority of their journey.
For most aircraft in service today, the satellite up/downlink connection is achieved with a mechanically steerable antenna mounted to the top of the fuselage. The antenna has a limited range of motion to maintain connection with the satellite while compensating for the movements of the aircraft during normal flight operation. These mechanical systems are now giving way to electronically steerable systems using phased array antennas to deliver more reliable connectivity with lower costs of operation and maintenance for the carriers.
Exploring the Fundamentals of Thin-Film Filter Technology in RF & Microwave Applications
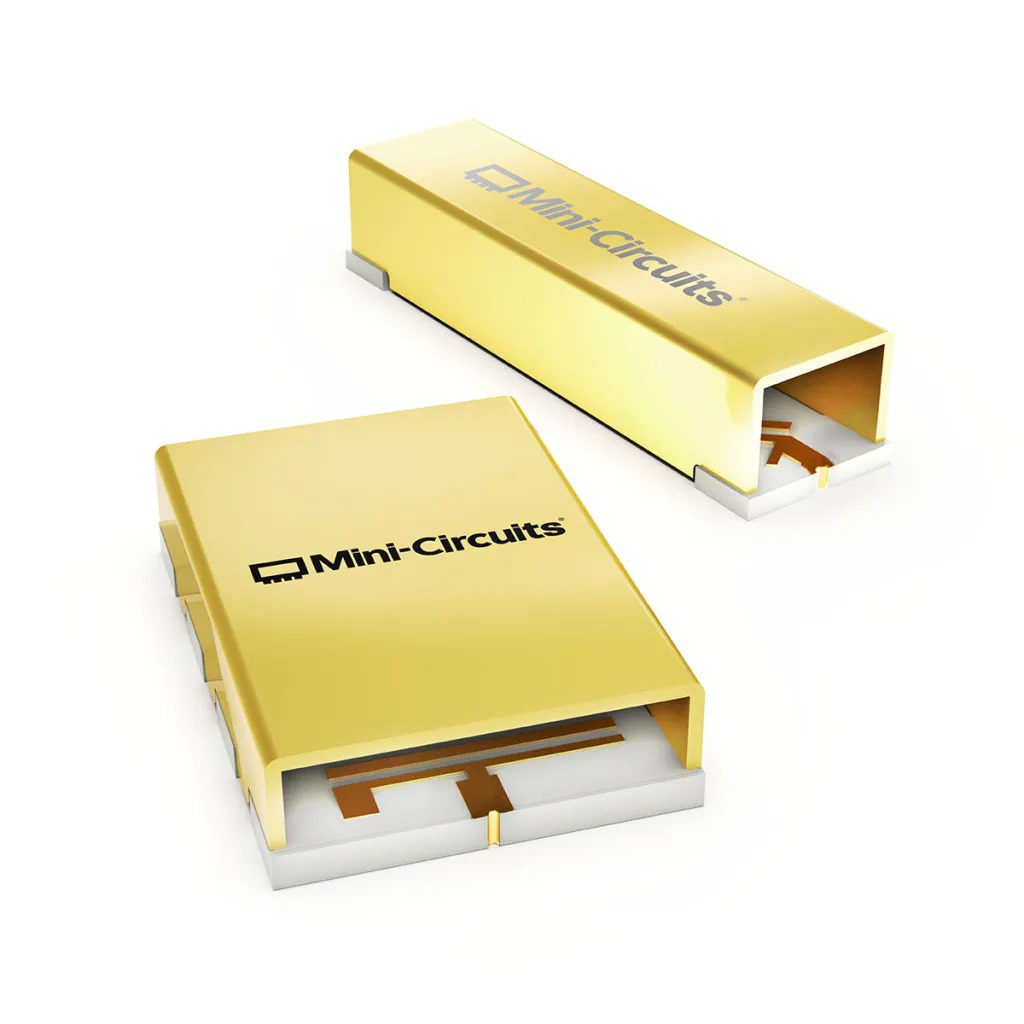
Finding the right filter for frequency ranges above the 3 GHz range is a perennial challenge for RF system engineers. Designers are typically looking for repeatable performance at production volume and a small, surface-mount form factor robust enough to withstand reflow onto their existing printed wiring board (PWB). Lumped element filters utilizing discrete wire-wound inductors and chip capacitors meet these criteria handily for passbands below about 3 GHz, but frequency response becomes more sensitive to variations in the physical structure of the device and temperature at higher frequencies, rendering this approach impractical.